Main Second Level Navigation
Breadcrumbs
- Home
- About
- News & Events
- News
- Tracing Single Brain Cancer Cells Simplifies Complexity Of Incurable Brain Cancers, Suggesting New Treatments, International Study Finds
Tracing Single Brain Cancer Cells Simplifies Complexity Of Incurable Brain Cancers, Suggesting New Treatments, International Study Finds
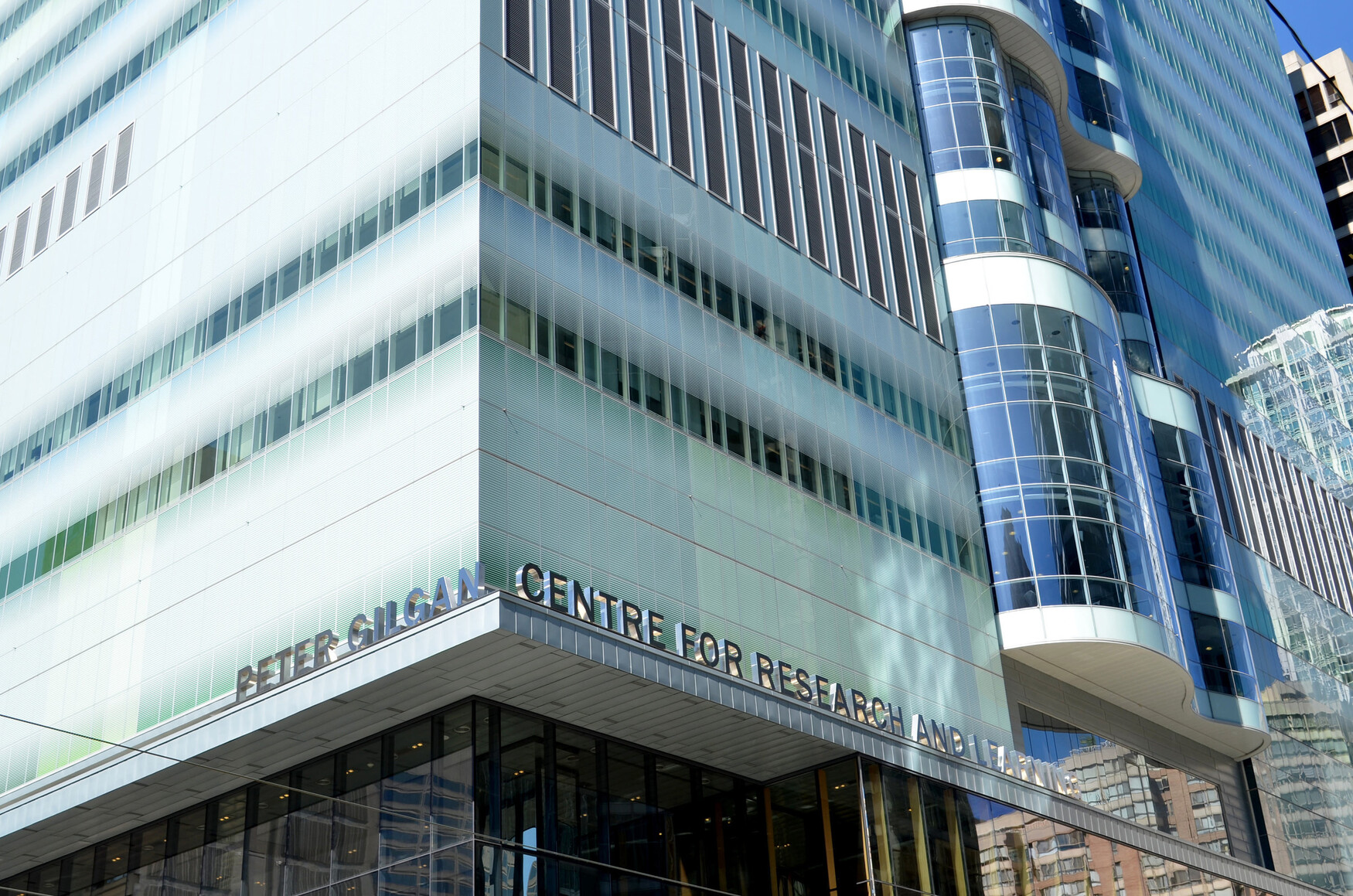
TORONTO – Using an innovative barcode-like system that tracks the behaviour of individual brain cancer cells, an international research team has gained a new understanding of how glioblastoma brain cancers grow and has identified potential new ways of treating these incurable cancers.
This novel approach enables scientists to track how each marked cell contributes to tumour growth, generating important new findings about how these cancers evade conventional treatments and invade normal brain tissue. The research, led by The Hospital for Sick Children (SickKids) and the University of Cambridge, is published in the Aug. 30 online edition of Nature.
Glioblastoma is a type of brain cancer that affects about 1,500 adults and 150 children in Canada every year, and is notorious for its complex genetics and poor response to all treatments, leading to death within about 15 months. While research into this devastating cancer has progressed, many aspects of the disease remain poorly understood, including simply how these tumours grow over time.
A collaborative team, including Dr. Connie Eaves from the University of British Columbia, dissected the functional properties of individual marked or “barcoded” cancer cells and their progeny – termed clones – which together form the evolving cancer mass.
“The approach we took is like the difference between looking at the final score in a sports match when it is over, to watching the game unfold in real time,” says the study’s co-principal investigator, Dr. Peter Dirks, Staff Neurosurgeon and Senior Scientist at SickKids. “Sometimes the final score doesn’t really tell us how the match unfolded, and this approach is like analyzing the individual performance of each player during the game. By neutralizing the star players, we can win over glioblastoma.”
Using this cell-tracking strategy, the research team found that only a few of the marked cells could give rise to long-term tumour growth, suggesting that a large portion of patient tumours contain cells that cannot multiply to make tumours grow. This finding is consistent with the stem cell model of cancer, initially proposed by Dr. John Dick at the University of Toronto in 1994, and the Dirks’ group in brain cancer in 2004, but established now with improved methods.
They then followed the mosaic of these cancer subpopulations through the processes of tumour growth, response to treatment and brain invasion. They found that glioblastoma is made up of many different clones (mini-cancers) which on the face of it might seem like a challenge for cancer therapy. However, using biophysical approaches, Professor Benjamin Simons and his team at Cambridge, jointly based at the Wellcome Trust-CRUK Gurdon Institute, the Wellcome Trust-MRC Cambridge Stem Cell Institute and the Cavendish Laboratory, Department of Physics, were able to show that the diversity of growth behaviours reflected a simple program of cell fate decision-making.
“We observed that the vast majority of clones retained a stereotyped pattern of growth, reminiscent of a stem cell “hierarchy”, where only a minority of stem cells within a clone underpin the growth of the clone. We designated clones with this pattern of growth as Group A,” says Dirks, who also holds a Garron Family Research Chair in Childhood Cancer Research and is Professor in the Departments of Surgery, Molecular Genetics, and Laboratory Medicine and Pathobiology at the University of Toronto. The team also identified other, rarer, more aggressive clones – labelled as Group B – that were able to escape this more organized pattern of growth.
“These results were surprising because glioblastoma is known to be a highly aggressive disease, with different tumour clones bearing a cocktail of different genetic mutations,” says Dirks. “Yet, when we analyzed each tumour cell’s behaviour individually, we found that the behaviour is highly structured, despite genetic differences between cancer cells within the same tumour, and is reminiscent of how human neural stem cells grow and give rise to mature brain cell types during normal development; this part was the most surprising.” However, he notes that the existence of the highly aggressive Group B subtype was actually not as surprising, given the complex genomics of the disease. “These clones were found to exist before therapy, but could be selected by therapy and could be a reason these tumours ultimately escape treatments. With the ability to single out clone types, we can now target how these Group B clones arise and grow.”
Building on these findings, the research team also identified two existing drugs that can slow the growth of glioblastoma tumour cells: one that acts on Group A cells and another that acts on Group B. These drugs target epigenetic processes, how the DNA is packaged, which determines which part of the cell’s DNA blueprint is active at any point in time.
“Our study suggests that drugs that can affect the behaviour of the abnormal glioblastoma stem cells could be very effective in treating glioblastoma,” says (Xiaoyang) Kevin Lan, who was a PhD student at the University of Toronto and the first author of the study. “Without the small population of cells that continuously sustain the tumour, our model predicts that the tumour could collapse. We think a strategy that targets both Group A and B cells could be highly effective. It suggests that we might also be able to side-step the need to target different tumour mutations, as it seems that multiple mutant forms follow the same behaviour. We need to target the hierarchy, the stemness form of the tumour, and there may be some common programs here, particularly in the way the DNA is specially packaged in stem cells to direct cell behaviour.”
Next steps for this research include using the cellular hierarchy model to test additional types of glioblastoma tumours in an effort to expand the scope of these findings. The team also plans to further characterize the molecular difference between the Group A and B cells to better target both types of cells with other drug treatments.
Funders for this study include the Canadian Institutes of Health Research, the Ontario Institute for Cancer Research, Stand Up To Cancer (SU2C) Canada and SickKids Foundation; and in the United Kingdom, research was funded by the Wellcome Trust with additional core support
from Cancer Research UK and the Medical Research Council.
This paper is an example of how SickKids is contributing to making Ontario Healthier, Wealthier and Smarter. www.healthierwealthiersmarter.ca.
About The Hospital for Sick Children
The Hospital for Sick Children (SickKids) is recognized as one of the world’s foremost paediatric health-care institutions and is Canada’s leading centre dedicated to advancing children’s health through the integration of patient care, research and education. Founded in 1875 and affiliated with the University of Toronto, SickKids is one of Canada’s most research-intensive hospitals and has generated discoveries that have helped children globally. Its mission is to provide the best in complex and specialized child and family-centred care; pioneer scientific and clinical advancements; share expertise; foster an academic environment that nurtures health-care professionals; and champion an accessible, comprehensive and sustainable child health system. SickKids is proud of its vision for Healthier Children. A Better World. For more information, please visit www.sickkids.ca. Follow us on Twitter (@SickKidsNews) and Instagram (@SickKidsToronto).
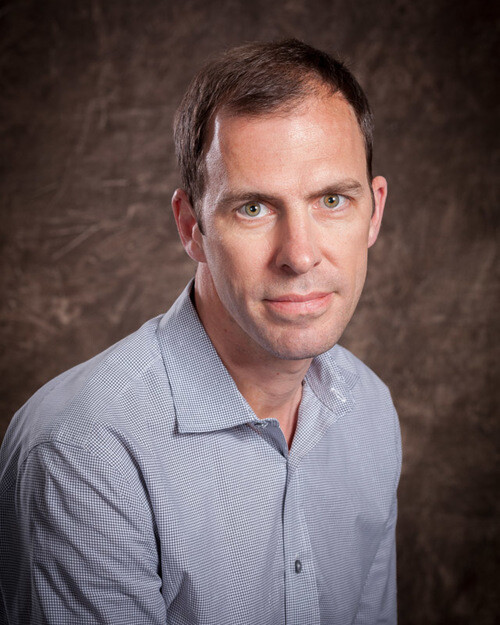
Media contacts:
*Suzanne Gold
The Hospital for Sick Children
416-813-7654, ext. 202059
*Caitlin Johannesson
The Hospital for Sick Children
416-813-7654, ext. 201436