Main Second Level Navigation
Breadcrumbs
- Home
- About
- News & Events
- News
- Field Spotlight: Cellular and Molecular Structure and Function
Field Spotlight: Cellular and Molecular Structure and Function
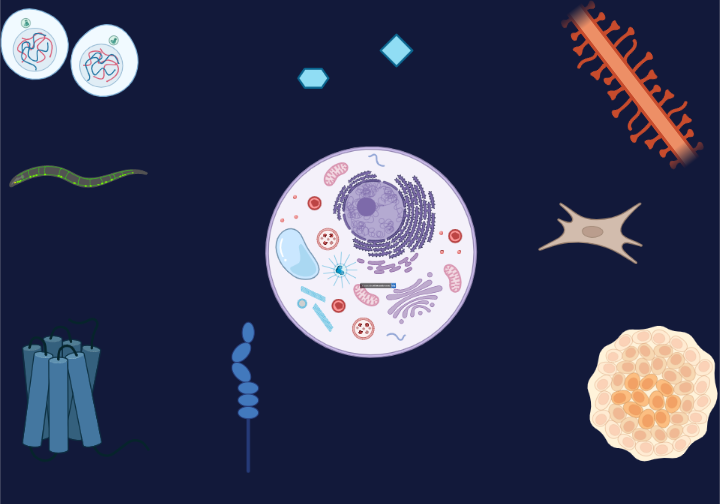
*Thank you to all those who donated and contributed material for this spotlight*
The Campos lab focuses on histones and chromatin biology. They study how histone chaperones deposit histones on DNA, and how that goes awry in disease. They are also interested in the contribution of histone proteins toward the transmission of epigenetic information during cell division. The group utilizes proteomic, in vitro, and cellular approaches to understand how histones and histone-binding proteins shape chromatin.'
The Collins lab specializes in the study of the fungus Neurospora mitochondrial plasmid, which encodes the VS ribozyme. The group’s previous work made significant breakthroughs in ribozyme research. This included identifying secondary structure elements in the VS ribozyme and characterizing the first long-range interaction in VS RNA (which form catalytically active conformations).
The Culotti lab conducts research into the nervous system development and function with the C. elegans model organism. Specifically, they search for genes and environmental factors that guide neuron growth cones and cell migration, with implications for several neurological disorders. The group co-discovered the remarkable similarities between the worm and human spinal cord growth, including similar molecules behind neuron guidance.
The Dennis lab specializes in cellular proteins called glycoproteins and their N-glycosylation, alongside their role in metabolism and disease. Their work demonstrated the role of glycoproteins in biological processes and conditions such as cancer and multiple sclerosis. Examples of their publications include demonstrating how N-glycan number and its degree of branching influence cell proliferation and differentiation and how Golgi apparatus N-glycan processing regulates/modifies cytokine receptors. Other examples include demonstrating the potential role of N-glycosylation dysregulation in MS and other autoimmune disorders and suppressing cancer cell proliferation by blocking the MGAT1 enzyme, which is used for branching N-glycans.
The Ernst lab investigates G protein-coupled receptor (GPCR) transmembrane signalling, which is essential for communication between the cell and its environment. One of their publications utilized DEER spectroscopy to comprehend how GPCRs utilize phosphorylation patterns to recruit arrestin proteins. The group also was the first to produce a crystal structure of the active form of human rhodopsin protein bound to an arrestin protein with X-ray lasers, providing insight into GPCR-mediated arrestin signalling.
The Kafri lab specializes in cell size and growth, alongside cell identity/individuality in cancer and developing systems pharmacology. Recently, they developed a pleomorphic (cells with variable size and shape) xenograph breast cancer tumour model in mice and developed multiple softwares that quantifies single cell responses and automatically classify fibroblasts based on morphological distinctions.
The Kaplan/Miller laboratory studies how neural stem cells are generated and maintained and communicate with their environment, how the environment and neurodevelopmental perturbations disturb normal stem cell function and discovering drugs that mobilize endogenous brain and skin stem and precursor cells for repair. We use proteomic and single cell transcriptomic and epigenomic analysis combined with lineage tracing and disease model mice to generate maps of how the different cell types communicate to control development and repair, and identify the ligands, receptors and signaling pathways and translational repressive mechanisms mediating these processes.
The Kay lab focuses on the NMR of supra-molecular machines and observing low populated, excited (aka invisible) states of proteins. The group creates novel NMR techniques that prevent loss of sensitivity with large systems such as the proteasome. This includes spin experiments that allow researchers to observe low populated or invisible states of proteins, as usually they can only see the most stable states and not higher-energy conformations (e.g. ligand binding, misfolding/unfolding, catalysis).
The Lavoie lab researches the molecular mechanisms behind chromosome segregation and transmission in eukaryotic cell division via the budding yeast model organism. Alterations of this process have been implicated in disorders such as trisomies in aborted fetuses and newborns and multiple types of cancer. Some of the group’s recent publications include demonstrating that cytokinesis requires suppression of actin networks via microtubules and that the CDK11p58 kinase is needed to regulate abscission during cell division in collaboration with the Wilde lab.
The Muffat lab generates tools incorporating human induced pluripotent stem cells to create new 3D cell cultures that allow observation of CNS cell types and their interactions. These methods essentially form a ‘disease-in-a-dish model’ replicating how natural CNS cells function in development, ageing and disease. There is a focus on the role of inflammation (such as viral infections) in neurological diseases such as Alzheimer’s, autism and MS. Dr. Muffat also holds the Canada Research Chair in Synthetic Neuroimmunology and Stem Cell Bioengineering.
The Okamoto lab specializes in the molecular mechanisms of the neural circuits behind learning, memory and other brain functions. The group established a novel technique and instrument which visualizes specific brain protein functions on a molecular level. They also characterized that the CaMKII protein is both a signalling and structural protein in the dendritic spine, which is involved in the brain’s synaptic network. The group plans to build an original microscope incorporating infrared lasers which can visualize and manipulate specific proteins simultaneously in real time.
The Protze lab implements human pluripotent stem cells to examine human heart development and disease with a focus on the heart’s pacemaker system. The lab has developed unique, developmental biology-based approaches for the differentiation of stem cells into pacemaker cells. To test the application and functional capacity of these pacemaker cells as biological pacemakers the lab is using in vitro assays alongside small and large animal models. In addition, the group also creates novel in vitro models using patient-specific, induced pluripotent stem cells to better understand the mechanisms of pacemaker diseases with the goal to develop better therapies for the future.
The Sicheri lab focuses on the structural biology of eukaryotic signalling proteins, specifically eukaryotic protein kinases and the ubiquitin proteasome system. Examples of their publications include uncovering the mechanisms and function of bacterial IpaH enzymes and the baculovirus PK2 protein. Others involved solving the X-ray crystal structure of the vaccinia virus protein K3L and conducting proteomic analysis of the tRNA modification KEOPS/EKC complex, which identified interactors for specific subcomplexes.
The Wilde lab aims to understand how cells divide to maintain a stable genome and prevent the accumulation of mutations that can lead to disease, in particular cancer and tissue specific defects found in numerous syndromes. Recent publications have focused on how different cytoskeletal elements remodel the cell to achieve successful cell division which have uncovered new pathways that open avenues to take novel approaches to study how cell migration and contractility become disrupted in a variety of disease states. Further work has discovered new cell cycle regulatory pathways that regulate the very final step in cell division to prevent aneuploidy.