Main Second Level Navigation
Breadcrumbs
- Home
- About
- News & Events
- News
- Field Spotlight: Genetic Models of Development and Disease
Field Spotlight: Genetic Models of Development and Disease
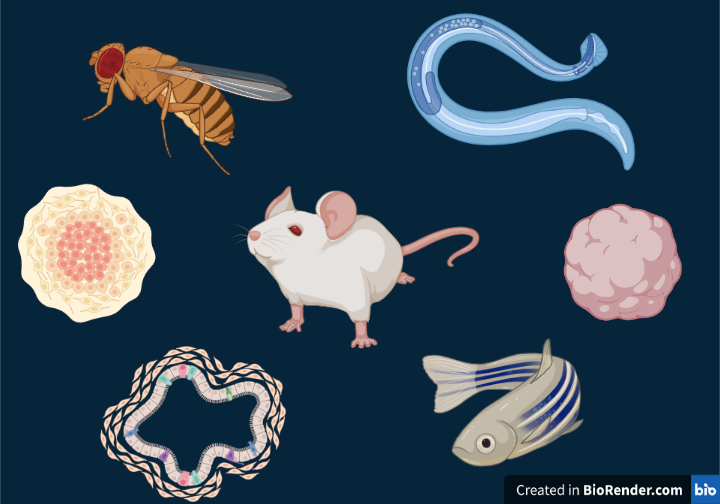
*Thank you to those who provided and contributed material for this content*
Many of our faculty employ model organisms to conduct ground-breaking research and make critical breakthroughs. Here are some standout accomplishments, publications and projects from our PIs specializing in this area:
The Andrews lab sheds light on the genetics of budding yeast and creates customized yeast collections. The group constructed the YETI collection, which allows induced transcription of engineered yeast genes on a single-gene level at their original loci. They have also developed yeast mutant model collections that can predict mutation effects and create a genotype to phenotype map based on different genetic contexts with CRISPR-based tools. Dr. Andrews is the Canada Research Chair in Systems Genetics and Cell Biology.
The Boulianne lab studies neurogenic genes and cell fates during neuronal development in Drosophila. The group developed fruit fly models of human ageing and disease such as ALS and Alzheimer’s. Some of their breakthroughs include characterizing a new component in the Notch signalling pathway that codes for an E3 ubiquitin ligase, finding new proteins involved in synaptic vesicle exocytosis and how altering SNARE protein levels changes the neurons’ ability for calcium-sensing. They also found that increasing SOD1 and Mgat1 expression in fly neurons increases lifespan significantly and that neuronal differentiation and Notch signalling require the presenilin protein.
The Brill lab uses the fruit fly Drosophila melanogaster to study the roles of the membrane lipids phosphatidylinositol phosphates (PIPs) via genetic methodologies. Some of their discoveries include unearthing previously unknown functions for PIPs and their pathway enzymes in developing sperm, maintaining tissue integrity and maturing secretory granules. One of their recent publications found that the Sac1 enzyme regulates fruit fly eye development by stabilizing microtubules and cell shape.
The Ciruna lab pioneered the first zebrafish models of adolescent idiopathic scoliosis (AIS). Examples of their breakthroughs include discovering how altering cerebrospinal fluid homeostasis, activating pro-inflammatory pathways and oxidative stress can play a role in abnormal spine development and curvature. Dr. Ciruna also holds the Canada Research Chair in Developmental Genetics and Cell Biology.
The Claycomb lab is currently conducting exciting projects with the C. elegans model organism to shed light on RNA interference, small RNAs (such as microRNAs) and epigenetics. Early studies from the lab made important insights on the CSR-1 Argonaute protein. Ongoing projects include investigating uncharacterized Argonaute proteins, mechanisms of the CSR-1 protein on chromatin and gene expression and extracellular communication with small RNAs. Dr. Claycomb is also the Canada Research Chair in Small RNA Biology.
The Cordes lab uses mouse models to investigate the impact of mutations on neural development (specifically the serotonergic system, which are neurons that produce serotonin) and animal behaviour, with implications on psychiatric disorders. With said mouse models, the team discovered the Gumby gene, which codes for a deubiquinating enzyme and regulates blood vessel growth in the blood-brain barrier. This finding makes the gene a potential cancer drug target through restricting blood flow to tumours and for Cri du chat syndrome as the gene’s locus is on chromosome 5, whose mutations is associated with patients. Dr. Cordes holds the Canada Research Chair in Molecular Mechanisms of Mood and Mind.
The Delgado-Olguin lab uses mouse models and cardiomyocytes to study a heart muscle disease called dilated cardiomyopathy (DCM) as well as cardiovascular development, placental maturation and the link between maternal obesity and heart disease. Some of their findings include how the G9a enzyme triggers the Notch pathway to regulate and mature the placenta and maternal obesity in mice alters cardiac progenitor transcription factors and increases heart disease risk. They’ve also found that the heart alters its energy production, or homeostasis, before it stops working.
The Derry lab utilizes C. elegans and human cells to understand mechanisms of organ development and disease. Current projects are focused on how oncogenic Ras/MAPK signalling is regulated by alternative polyadenylation, biological tube development, the molecular basis of the neurovascular disease Cerebral Cavernous Malformations (CCM), and protein-based mechanisms of transgenerational inheritance. Dr. Derry also holds the Canada Research Chair in Animal Models of Human Disease.
The Dick lab pioneered development of immune-deficient mice to gain insight into normal and leukemic human blood stem cells. He also produced the first xenograft models of human leukemia, now the global gold standard for normal and leukemic stem cell detection. Their current projects revolve around utilizing multi-omic single cell analyses and genetic tools to determine the molecular basis of stemness and how it goes awry in leukemogenesis. Dr. Dick is also the Canada Research Chair in Stem Cell Biology.
The Egan lab uses gene targeting and chromosome engineering to generate immune competent mouse models of human breast cancer. Examples include genetically engineered mouse models (GEMM) which mimic Immune Related Invasive Lobular Carcinoma (IR-ILC) and models for Lobular B Ductal Carcinoma of the breast, as well as models for metastatic recurrent ILC. These mice are then studied and exploited for the development of immune-based combination therapy. Finally, the lab also utilizes Sleeping Beauty transposon mutagenesis and Apobec3 systems to study mammary tumor evolution and progression.
The Ellis lab tinkers with induced pluripotent stem cells (iPSCs) for modelling human disease and testing potential drugs. The group utilizes a new EOS reporter vector that rewires patient-derived fibroblasts into iPSCs and CRISPR editing to make isogenic cell pairs. They’ve used these systems to replicate cell phenotypes and expression patterns to model Rett syndrome, autism and pediatric cardiomytopathies.
The Fraser lab takes advantage of C. elegans to screen and identify potential anti-parasitic drugs against helminths, or worms. This group constructed their own imaging platform to exactly measure drug impact on worm movement and how worms react to drug administration. The platform facilitates researchers to simultaneously measure worm movement every 0.5 seconds and movement of 2000 worms and screen for mutants with differing drug responses.
The Hopyan lab focuses on biophysical mechanisms of morphogenesis of the mouse embryo. The interdisciplinary lab combines genetic, physical and computational methods to define how cell movements generate organ primordia such as the limb bud and craniofacial structures. They developed tools to image cell movements in live embryos, measure viscoelastic properties of tissues, forces generated by cells, and voltage gradients within tissues. The lab is currently pursuing concepts such as phase transition, durotaxis, galvanotaxis, and thermodynamic regulation of morphogenetic movements.
The Huang lab studies physical properties and mechano-electrical-chemical signaling in cancer. They have made pivotal breakthroughs in uncovering the role of ion channels in brain cancer. For example, they determined a mechanism behind why brain tumour tissue is stiffer than its healthy counterpart and how this discovery can unearth a way to slow tumour development. Specifically, they found that an ion channel called PIEZO1 detects the pressure resulting from the stiffening and opens as a result, causing tumour growth. The group incorporates methods such as Drosophila, mouse and xenograft models in their research. Dr. Huang also holds the Canada Research Chair in Cancer Biophysics.
The Hui lab studies mouse models to discern the function of hedgehog signalling and Iroquois homeobox genes in development and diseases such as cancer, obesity and heart failure. The group demonstrated that three Gli transcription factors play a role in mammalian Hedgehog signalling and how precise timing of Hedgehog activation is needed for normal limb development. Other studies demonstrated how mouse Iroquois box (Irx) genes have overlapping and unique functions in embryonic patterning and terminal differentiation, and that Irx3 is required for mouse energy homeostasis.
The Hurd lab investigates mitochondrial biology using Drosophila and mammalian model systems such as human induced pluripotent stem cells. Specifically, they aim to understand how the female germline removes harmful mutations from mitochondrial DNA and how the mitochondria impact stem cell differentiation. One of the lab’s publications found how fragmenting the mitochondria pinpoints and facilitates the removal of mutant mtDNA genomes from the germline.
The Kim lab uses mouse models and genetics alongside stem cell organoid culture to study how gut stem cell niches impact development and diseases such as IBD, peptic ulcers and cancer. One of the group’s publications established a new mouse model with a mutant ARID1A gene that demonstrates how said mutation promotes gastric tumour progression and potential therapeutic cocktail combining a p53 agonist Nutlin-3 and epigenetic inhibitor TP064. They have also made important insights in the cellular basis of IBD and the role of Hedgehog signalling in stem-cell-derived beta cells (which produce insulin).
The Krause lab is currently investigating how long noncoding RNAs take part in male reproduction using the model organism Drosophila. During sperm formation/spermatogenesis, chromatin opening, and accessibility resulted in enhanced expression and evolution of lncRNA genes. These genes seem to have evolved relatively recently and appear to be responsible for the increased transposable element activity. The group discovered hundreds of roles for these genes in sperm production and activity. They analyzed lncRNA expression patterns on a genome-wide level in Drosophila reproductive tissues, subsequently examining where the cellular and subcellular lncRNAs localized and distributed to perform genetic and molecular assessments and determine possible functions.
The Lefebvre lab leverages mouse models to study developmental neurobiology and how neurons become neural circuits. One area of focus is using transgenic mice to investigate clustered protocadherins (cPcdhs) in the retina and brain. An example of the group’s work is analyzing the interactions between multiple Pcdh clusters and finding its essentiality in neural development, specifically neuron survival and dendritic separation or self-avoidance. Another aim is studying how neuron connections lead to retinal degeneration and ultimately blindness in mice. Dr. Lefebvre also holds the Canada Research Chair in Developmental Neural Circuitry.
The Li lab employs pluripotent stem cells and 3-D organoids to examine human brain formation and development and how neurological disorders such as autism and epilepsy alter it. One of their publications modelled microcephaly in human brain organoids that overexpressed the PTEN protein and found that increased PTEN dosage alters normal brain development.
The Lipshitz lab studies post-transcriptional regulation of gene expression in development and disease. The focus is on the role of RNA-binding proteins in controlling mRNA stability, translation and subcellular localization. First, the lab studies post-transcriptional regulation during the maternal-to-zygotic transition in early embryos as well as in neural lineages in the developing brain of the fruit fly, Drosophila melanogaster, using diverse techniques including genetics, genomics, transcriptomics, proteomics and computational biology. Second, Drosophila is used to understand the mechanisms underlying rare human diseases caused by mutations in RNA-binding proteins. Finally, the lab has initiated a study of the role of RNA-binding proteins in autism spectrum disorder by producing phage-displayed synthetic antibodies that recognize dozens of these proteins that have been implicated in the disease and using the antibodies to study post-transcriptional regulation of the developmental transcriptome of human pluripotent stem cells induced to differentiate into neurons.
The Osborne lab uses mouse models to study neurodevelopmental disorders caused by deletions and duplications in human chromosome 7q11.23, specifically Williams and Dup7 syndromes. The group created mouse models and induced pluripotent stem cell lines that either has a deletion or duplication of 25 genes on chromosome 7. The lab aided in defining the structure of this genomic region and unearthing the mechanism behind the deleted region by discovering an inversion polymorphism increasing the risk of a deletion/duplication. They also use mouse models to study the epigenetics of Williams and Dup7 syndromes.
The Park lab leverages mouse models to gain insight on neurodegenerative disorders, with a focus on ALS. The group created novel mouse and fruit fly models with mutated MATR3 S85C, which mimics the early stages of human ALS. They found that axonal transport defects may play a role in pathogenesis and loss-of-function in MATR2 results in neuronal defects in specific types.
The Pelletier lab is interested in understanding the molecular mechanisms underpinning centrosome and cilia biogenesis and function in human cells and their link to human diseases. To do this they use mammalian cell culture, organoids and mouse models to shed light on the role of centrosomes and cilia in cell cycle control, mitotic progression, and cancer cell motility, migration and metastasis. The group defined an evolutionary conserved pathway regulating centriole duplication (component of centrosome) and charted the protein interaction landscape of the centrosome-cilium interface. Dr. Pelletier holds the Canada Research Chair in Centrosome Biogenesis and Function.
The Scott lab employs zebrafish to research heart development and regeneration. The group discovered that embryonic heart development processes get retriggered during an adult zebrafish’s heart regeneration. Their work was also the first to describe how the zebrafish heart develops through two cell waves and demonstrated the essentiality of the Apelin receptor GPCR (Aplnr) in heart development. Additionally, the group discovered several enhancers that are activated during heart injury and made mutant and transgenic zebrafish lines based on them, alongside mutant zebrafish modelling Cerebral Cavernous Malformations (CCMs).
The Smibert lab makes use of the Drosophila model system to study post-transcriptional regulation of gene expression. The group also aims to map out the ribonome (aka all RNA/protein interactions) of the Drosophila embryo and the function of the Smaug protein in the embryo. They have also demonstrated that the Smaug protein inhibits translation and triggers the degradation of hundreds of early embryonic mRNAs and how this protein regulates its targets.
The Spence lab focuses on sex-determining and other developmental genes in C. elegans. One of their accomplishments includes explaining a new type of epigenetic regulation dependent on maternal RNA to regulate the next generation’s germline gene expression. Examples of their work include finding that proteolysis of the sex-determining protein TRA-1A is specific to male worms and that the CUL-2 ubiquitin ligase modulates TRA-1 through degradation, allowing for male phenotypes.
The van der Kooy lab employs a wide range of model organisms in their research. Firstly, the group leverages C. elegans and mice to analyze genes involved in learning and memory. Additionally, they utilize mouse models to study the neurobiology of motivation (specifically in the context of psychoactive drugs such as opiates), neural development and stem cell biology (specifically neural, retinal and pancreatic stem cells). The group was the first to describe stem cells in an adult mammal eye and isolated a rare stem cell type from mouse and human pancreas.
The Wrana lab utilizes human organoid and mouse genetic models to study the role of extracellular signalling in regulating cell behaviour, in the contexts of cancer and development. Dr. Wrana and his group were the first to discover the TGF-beta signalling pathway, the Smurf ubiquitin ligase family and how both collaborate with the Hippo tissue size control pathway. All these findings made critical breakthroughs in comprehending cancer initiation and progression.
The Zhen lab uses mouse and C. elegans models to shed light on the nervous system. Some of the lab's breakthroughs include the creation of a RoboWorm, whose movement is controlled by light or optogenetics and being the first to deduce how new neural connections are made in the brain from birth to adulthood. The group also generated fluorescent markers to visualize structures in live C. elegans and discovered novel genes used for synapse development, with implications for psychiatric disorders. Dr. Zhen also holds the Canada Research Chair in Neural Circuit Development and Function.